While the perturbations resisted in a control relation can originate either inside (e.g. functioning errors or quantum fluctuations) or outside of the system (e.g. attack by a predator or changes in the weather), functionally we can treat them as if they all come from the same, external source. To achieve its goal in spite of such perturbations, the system must have a way to block their effect on its essential variables. There are three fundamental methods to achieve such regulation: buffering, feedback and feedforward (see Fig. 1).
Buffering: absorbing perturbations
Buffering is the passive absorption or damping of perturbations. For example, the wall of a thermostatically controlled room is a buffer: the thicker or the better insulated it is, the less effect fluctuations in outside temperature will have on the inside temperature. Other examples are the shock-absorbers in a car, and a reservoir, which provides a regular water supply in spite of variations in rain fall. The mechanism of buffering is similar to that of a stable equilibrium: dissipating perturbations without active intervention. The disadvantage is that it can only dampen the effects of uncoordinated fluctuations; it cannot systematically drive the system to a non-equilibrium state, or even keep it there. For example, however well-insulated, a wall alone cannot maintain the room at a temperature higher than the average outside temperature.
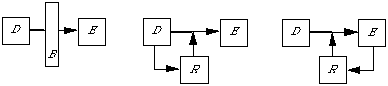
Fig. 1: basic mechanisms of regulation, from left to right: buffering, feedforward and feedback. In each case, the effect of disturbances D on the essential variables E is reduced, either by a passive buffer B, or by an active regulator R.
Feedforward: anticipating perturbations
Feedback and feedforward both require action on the part of the system, to suppress or compensate the effect of the fluctuation. For example, a thermostat will counteract a drop in temperature by switching on the heating. Feedforward control will suppress the disturbance before it has had the chance to affect the system's essential variables. This requires the capacity to anticipate the effect of perturbations on the system's goal. Otherwise the system would not know which external fluctuations to consider as perturbations, or how to effectively compensate their influence before it affects the system. This requires that the control system be able to gather early information about these fluctuations.
For example, feedforward control might be applied to the thermostatically controlled room by installing a temperature sensor outside of the room, which would warn the thermostat about a drop in the outside temperature, so that it could start heating before this would affect the inside temperature. In many cases, such advance warning is difficult to implement, or simply unreliable. For example, the thermostat might start heating the room, anticipating the effect of outside cooling, without being aware that at the same time someone in the room switched on the oven, producing more than enough heat to offset the drop in outside temperature. No sensor or anticipation can ever provide complete information about the future effects of an infinite variety of possible perturbations, and therefore feedforward control is bound to make mistakes. With a good control system, the resulting errors may be few, but the problem is that they will accumulate in the long run, eventually destroying the system.
Feedback: correcting perturbations after the fact
The only way to avoid this accumulation is to use feedback, that is, compensate an error or deviation from the goal after it has happened. Thus feedback control is also called error-controlled regulation, since the error is used to determine the control action, as with the thermostat which samples the temperature inside the room, switching on the heating whenever that temperature reading drops lower than a certain reference point from the goal temperature. The disadvantage of feedback control is that it first must allow a deviation or error to appear before it can take action, since otherwise it would not know which action to take. Therefore, feedback control is by definition imperfect, whereas feedforward could in principle, but not in practice, be made error-free.
The reason feedback control can still be very effective is continuity: deviations from the goal usually do not appear at once, they tend to increase slowly, giving the controller the chance to intervene at an early stage when the deviation is still small. For example, a sensitive thermostat may start heating as soon as the temperature has dropped one tenth of a degree below the goal temperature. As soon as the temperature has again reached the goal, the thermostat switches off the heating, thus keeping the temperature within a very limited range. This very precise adaptation explains why thermostats in general do not need outside sensors, and can work purely in feedback mode. Feedforward is still necessary in those cases where perturbations are either discontinuous, or develop so quickly that any feedback reaction would come too late. For example, if you see someone pointing a gun in your direction, you would better move out of the line of fire immediately, instead of waiting until you feel the bullet making contact with your skin.
Reference:
Heylighen F. & Joslyn C. (2001): "Cybernetics and Second Order Cybernetics", in: R.A. Meyers (ed.), Encyclopedia of Physical Science & Technology , Vol. 4 (3rd ed.), (Academic Press, New York), p. 155-170